Physics often gives us absolute limits that engineers and physicists find hard to reach. Examples include Absolute Zero, the coldest possible temperature which we’ve gotten closer and closer to in the lab but haven’t quite attained yet. There’s also the cosmic speed limit c . Our particle accelerators can accelerate particles to 99.999… % of the speed of light, but we know that we can’t make a particle with mass reach the speed of light (299,792,458 m/s in vacuum).
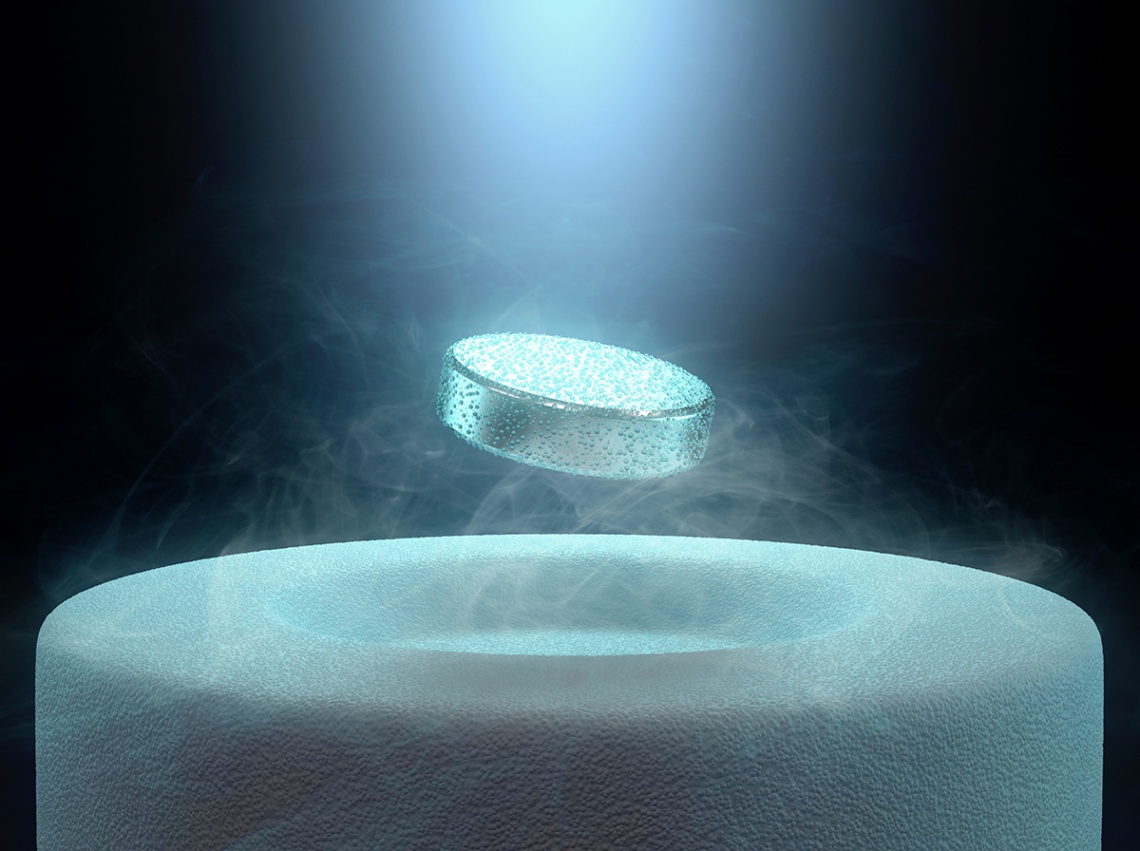
Therefore it comes as a bit of a surprise that superconductivity – the property of a substance having absolutely zero electrical resistance not only exists, but has even found widespread applications in MRI machines and superconducting magnets for particle accelerators. The catch is that these machines have to be cooled and kept cool at very low temperatures, around the boiling point of liquid nitrogen (77 K). What we’re really after is a room temperature superconductor, and a team of scientists in India may have found it. But before I tell you that story, we have to look back at things a bit.
The History
The 1900s were an interesting time for physics. While we knew that the resistance of a metal increased with increasing temperature, what happened when a metal was cooled to near absolute zero was unclear.
1.) Augustus Mattheissen believed that the resistance would go on decreasing till the value reached a constant, non zero value at 0K.
2.) Others such as William Thompson (Lord Kelvin) believed that electrons would stop moving at absolute zero and thus resistance would tend to infinity.
3.) While still others (such as Heike Kamerleigh Onnes) believed that the resistance would drop to zero at absolute zero.
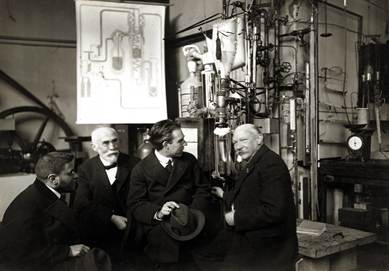
Onnes was a professor of experimental physics at the university of Leiden, and founded one of the best cryogenic laboratories in the world. Infact, The coldest temperature on earth was achieved in this very laboratory when Onnes managed to liquefy Helium (which has a boiling point of 4.2K) in 1908.
When he checked the conductivity of many pure metals, he observed that the resistance of mercury dropped to zero. He named this phenomena superconductivity, and would later go on to win a Nobel prize for it.
Later scientists such as Meissner would go on to find out that there were other properties associated with superconductors, such as the expelling of all magnetic fields. This effect is the reason for magnets levitating over superconductors.
The Bardeen Cooper Schrieffer (BCS) Theory
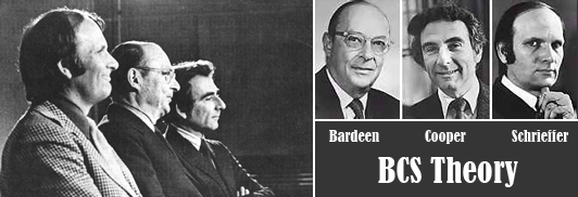
The properties of superconductors (well, at least low temperature ones) can be best explained by the BCS theory. If the name Bardeen sounds familiar to you, it is, he’s the same person who was on the team that invented the first working transistor and is the only person to win two Nobel prizes in physics.
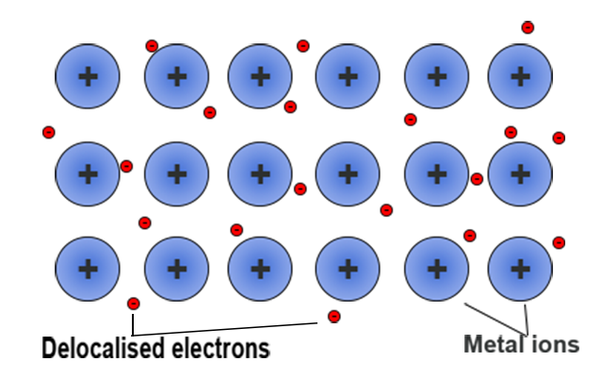
Metals normally conduct because there is a delocalized sea of electrons. When a potential is applied across the ends of a wire – for example with a battery the electrons begin to move in one direction. The electrons face opposition from Nuclei (protons and neutrons). They collide with the nuclei, which in turn causes the electrons to lose energy (and change direction) and makes the nuclei vibrate even more. The higher the temperature is, the more energy present within the atoms which is why the resistance increases.
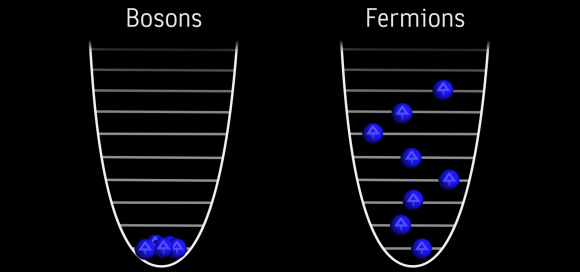
Electrons, Protons and Neutrons are all particles known as Fermions. One important property of these Fermions are that they cannot exist in the same state (in other words, they must obey the Pauli exclusion principle). You may have seen models of an atom where different electrons occupy different positions in space around the nucleus. These electrons don’t occupy the same space because of the Pauli exclusion principle, the obedience of this principle is so absolute that Neutron stars – some of the densest objects in the periodic table are prevented from further collapse because neutrons cannot be in the same state!
Now, we can finally give a rough- hand wavey explanation of BCS theory . The actual proof involves a fair bit of QM and mathematics, but as a first approximation the below visualization should do.
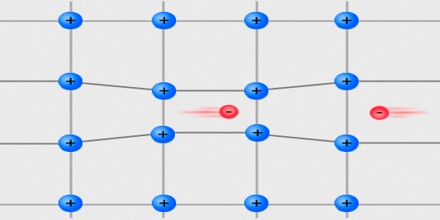
When metals are cooled down to near absolute zero, the movements in the metal lattice decrease significantly, An electron in the lattice being negatively charged attracts other nuclei towards it, and hence the region around the electron becomes slightly more positively charged than its surroundings. Other electrons in the metal are attracted to this distortion in the lattice, which is called a Phonon. Two of these electrons form what is known as a Cooper pair.
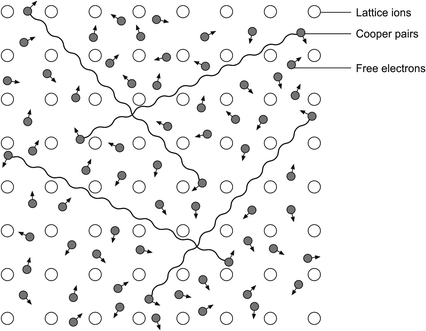
Now, while individual electrons are Fermions, pairs of electrons can act as particles known as Bosons. An example of a Boson is a photon (particle of light). Unlike Fermions, Bosons do not have to obey the Pauli exclusion principle. The entire system can collapse down to the lowest energy state! Many Cooper pairs form in the metal lattice, overlapping and as the electrons move in the direction of applied current they drag the electrons of the Cooper pairs along with them. Since all the electrons are in their lowest state, energy cannot be extracted from the system and the material becomes a superconductor! The electrons form what is known as a Bose Einstein condensate within the confines of the metal.
The Bose Einstein Condensate is a special state of matter predicted by SN Bose and Albert Einstein. Very crudely, the wave nature of matter dominates at very low temperature and all the particles act in unison. BEC has been associated with other properties too (such as superfluid helium flowing without any resistance).
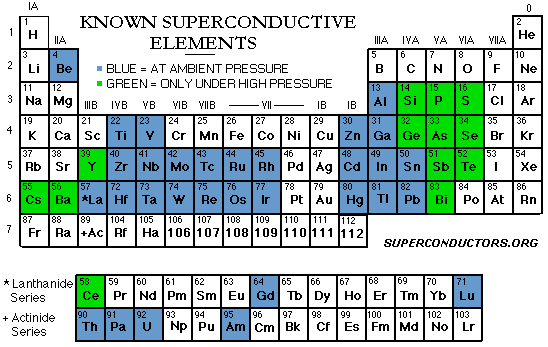
The BCS theory explains the properties of low temperature superconductors (below 30K) really well but fails at higher temperatures. This does not mean that BCS theory cannot explain all kinds of high temperature superconductivity- indeed some insane superconductors such as H2S at 203K and theorized metallic hydrogen at the core of Jupiter are proposed to be BCS superconductors. But the catch is that both these superconductors only become superconductors at very high pressures. This is not desirable at all since maintaining high pressure is not easy.
High Temperature Superconductivity
Following Onnes discovery, several compounds of Niobium such as NbTi and Nb3Sn were found to superconduct all the way till 20K. While this was an improvement, such low temperatures meant that liquid helium had to be used, which is prohibitively expensive.
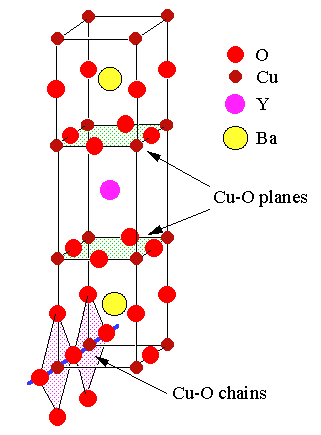
In 1986, a team at an IBM research lab in Zurich, Switzerland found superconductivity in a Barium doped compound of Lanthanum and copper oxide which was found to superconduct at 35K. Several copper based ceramics known as Cuprates would later be found, starting with YBa2Cu3Ox which was the first superconductor with a critical temperature (temperature below which a substance acts as a superconductor) of 77K. This is important because it allowed liquid nitrogen to be used, which is cheap and can be made on site.
The highest temperature in Cuprates we have seen till now is 133K, in a compound known as Mercury Barium Calcium Copper Oxide ( HgBa2Cu2Ca3O8). Cuprates have a structure known as perovskite – which we suspect has something to do with their superconducting. Earlier the only kind of high temperature superconductors known were cuprates – so much so that we thought that there might be some property of Copper that causes high temperature superconductivity!
This was found to be false when Iron based high temperature superconductors were found in 2006. Currently these compounds are being closely studied to hopefully find a working theory for non BCS superconductivity.
There are many theories for why room temperature superconductors work with varying degrees of success, and as of 2019 we do not have one theory that explains all the aspects satisfactorily. It is suspected that high temperature superconductivity involves genuine electronic interactions unlike the electron-phonon interactions of BCS superconductors.
Type I and Type II superconductors
Superconductors are sensitive to magnetic fields – apply a large enough magnetic field and any superconductor will break down. How this breakdown occurs is what determines if a material is a type I or type II superconductor.
Type I superconductors are usually pure metals (like the periodic table we saw earlier). In these, magnetic fields cannot penetrate the bulk of the metal at all – they exhibit the Meissner effect almost completely. The moment a field stronger than the critical field Tc is applied, superconductivity breaks down completely and this happens at a low value of less than 1 Tesla.
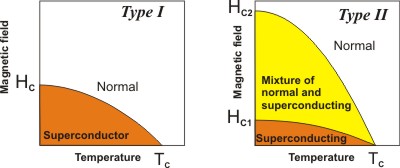
Type II superconductors are the alloys and ceramics. Things are more flexible here, initially they allow magnetic fields to pass through and it leads to the creation of magnetic vortices, this happens at magnetic field value Tc1. The vortices get larger and larger until at a value of Tc2 the superconductivity is destroyed.
The amount of magnetic fields a superconductor can tolerate is very important, as passing a current in the superconductor sets up a magnetic field in it, and thus if too much current flows through, superconductivity is destroyed. This can severely limit the usefulness of type I superconductors.
Why is superconductivity important?
Human energy consumption has been steadily increasing, and a whole lot of energy that we produce is lost due to transmission losses in power lines. All of our electronics produce waste heat due to resistance too. Superconductors could save materials by allowing really thin transmission lines, and would cut out the energy losses entirely. Even with the expense of cooling, superconductors have made into devices such as MRI machines, superconducting magnets for particle accelerators (such as the Large Hadron Collider) and SQUID magnetometers.
To be fair, just having a room temperature superconductor is not enough. It must have favorable properties for our use which include:
1.) The material should be ductile, in other words, we should be able to make wires out of the material. While conventional BCS superconductors are great for this (they are metals, of course they are ductile!) , the ceramics which superconduct at higher temperatures fare badly at this department because ceramics are rather brittle and prone to breaking.
2.) The superconductivity of the material should not be destroyed by the application of a large current – This can be a problem with the low temperature superconductors. The current sets up a magnetic field which can destroy superconductivity. Although some significant progress has been made in this area. Some of our superconductors can withstand upto 100s of Teslas.
3.) Superconductors are sensitive to changing electric fields, which makes them unsuitable for applications involving AC current.
Currents set up in superconductors stay there forever unless removed. Room temperature superconductors would mean stuff like super efficient Maglev trains, computers that can be a lot faster than what we have today, as one of the things that limits computer speed today is heat produced by resistance in the components.
The IISc findings – Anshu Pandey and Dev Kumar Thapa
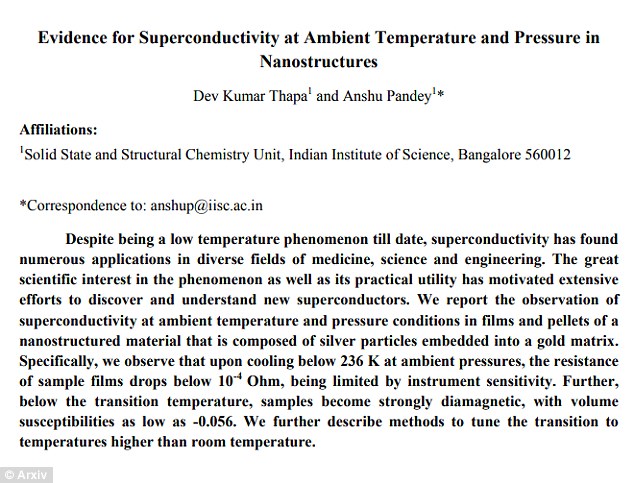
Now we get to the crux of the issue. Superconductivity as high as 250K has been achieved but at very high pressures, and for atmospheric pressures and temperatures the cuprates still hold the record. The Cuprates are still more than 100K away from 273K (above zero degrees Celsius).
From time to time various claims have been made on room temperature superconductivity, we will focus on the latest such claim which has gathered a lot of attention worldwide which is the finding made at a lab in 2018 at the Indian Institute of Science, Bengaluru in 2018 and the follow up paper published by the authors in 2019.
On July 23rd 2018, Anshu Pandey, an associate professor from the solid state lab of Indian Institute of science and his student Dev Kumar Thapa published a preprint to ArXiv. Initially it was met with lukewarm response by the media, with not much other than articles by the Hindu and the Wire covering it.
The scientific community though, was livid. The paper had a few oddities that immediately stood out – the scientists claimed to have used a Au-Ag (Gold-Silver) mixture. This is strange because gold and silver are one of the few metals known to not superconduct in bulk form, so it would be strange for the colloidal form of the same material to exhibit these properties. Moreover, how the authors exactly created this mixture was not detailed. The authors silence frustrated the scientific community, with many of them feeling that since the authors had made the paper public, they were answerable to the scientific community.
In India, A scientist from the Tata Institute of Fundamental Research (TIFR) Prof Pratap Raychaudhuri led the discussions on facebook, calling upon the authors to make more details available.
The news of the Indian discovery had travelled around the world, and a Professor from MIT, Dr Brian Skinner was interested in the details. Zooming into the graph, he noticed a discrepancy in a graph. The levels of noise in the data was low for higher temperatures, and more for lower temperature, which was definitely unusual. This was troublesome, as it implied fraud, not just an innocent mistake. It was here that things would begin to take a strange turn.
The curious case of the Imposter
Prof Raychaudhuri details in his facebook post that he recieved an email from TV Ramakrishnan, who is an acclaimed scientist, asking him “Not to criticize Pandey and Thapa so harshly”. Ofcourse, Scientists usually never mince words when it comes to criticism of sloppy work, so Prof Raychaudhuri did not take kindly to this. He wrote a sharp letter to Prof TVR, who later called him up and claimed that he had not written the email at all.
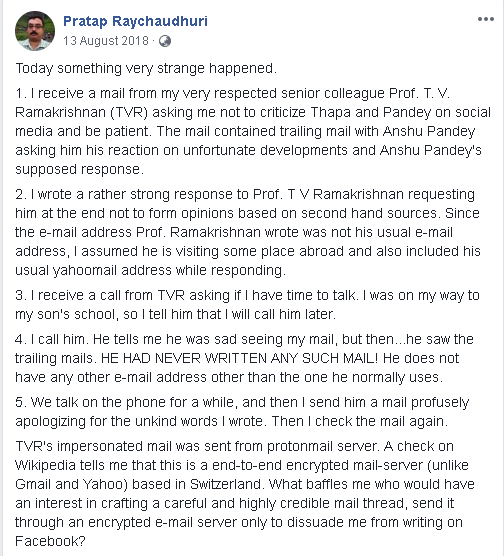
The email was carefully crafted with trailing emails from Anshu Pandey himself, and the email id was from one Wiles Licher on a protonmail server. Protonmail is an email client based in Switzerland that offers an encrypted email service, so tracking down who this Wiles Licher was was impossible.
Meanwhile, Brian Skinner also received a friend request from an account named Wiles Licher, which was only a month old, and had photos of Julius Caesar with one liners. The account was later deleted.
Who was this character? A disgruntled student? some random internet troll? perhaps we will never know. Dr Skinner would later go on to receive a final email from the imposter.
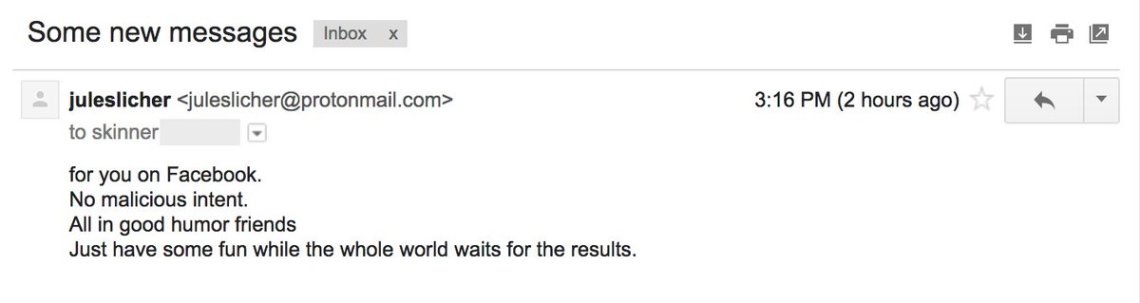
The Update and new sources of confusion.
Many scientists had begun working on the problem of the noise that Brian Skinner had outlined, Prof Raychaudhuri had himself detailed one such possible mechanism, although others remain skeptical. The authors concur that the strange patterns of noise in the graph might actually be some new scientific phenomenon.
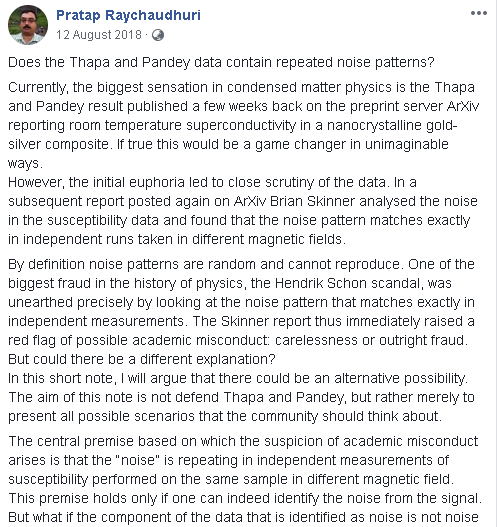
Almost a year later, on 21st May 2019, the authors finally posted a much expanded version of their paper. The paper now paid more emphasis on experimental rigour, much to the relief of those involved. The team had also expanded, with many additional researchers.
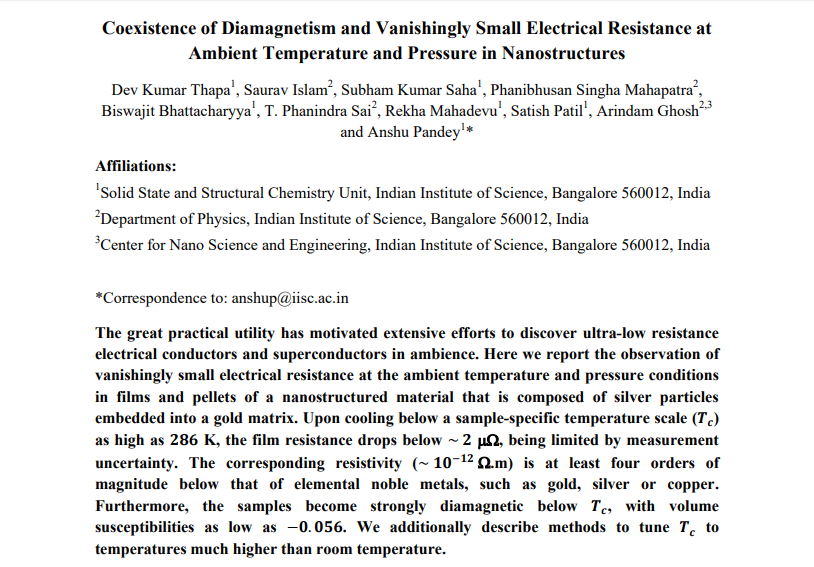
The Hindu noted in its 2019 article:
Prof. G. Baskaran, a SERB Distinguished Fellow at The Institute of Mathematical Sciences, who works on the theory of superconductivity, was quick to provide a theory soon after the preprint was posted in 2018. In his theory, monovalent character of silver and gold and repulsion among electrons could produce room temperature superconductivity under certain restrictive conditions. Prof. Baskaran is excited that the Thapa-Pandey system precisely provides such conditions. “This looks like a case where granular superconductors play a role. I am excited that the key first step in this challenging field has been brought about by a systematic and detailed effort.”
The new paper had details on how to prepare the said compound, and the authors had not backed down from their earlier claim. They said that they had tested 140 samples and found evidence for superconductivity in 10 of them. They also uploaded video evidence on YouTube
The supplementary section of the paper notes that the chemicals were sourced from Sigma Aldrich at 99% purity, and that the samples that showed superconductivity were only from specific lot numbers. The matter is outlined in this reddit thread. This could mean that the 1% impurity present in the samples may be the reason for the superconductivity. The authors say that they have finally submitted the paper to a journal, and the matter is still under investigation.
To conclude, we’ll end with Brian Skinner’s tweet:
Guys, it’s hard to properly convey how surreal this all feels. It’s way more drama than I ever thought I would encounter in physics.
Thank you for reading.
Footnotes:
1.) https://www.deccanherald.com/city/iisc-scientists-revise-superconductivity-paper-736797.html
2.) https://en.wikipedia.org/wiki/High-temperature_superconductors
3.) https://en.wikipedia.org/wiki/John_Bardeen
4.) https://en.wikipedia.org/wiki/Technological_applications_of_superconductivity
5.) http://www.supraconductivite.fr/en/index.php?p=applications-intro
6.) http://www.superconductorweek.com/what-is-superconductivity
7.) https://arxiv.org/abs/1409.4673
9.) https://arxiv.org/abs/1412.0460
10.) https://jqi.umd.edu/glossary/bardeen-cooper-schrieffer-bcs-theory-superconductivity
12.) https://en.wikipedia.org/wiki/Boson
13.) https://www.nature.com/articles/d41586-018-06023-x
14.) https://en.wikipedia.org/wiki/Heike_Kamerlingh_Onnes
15.) https://en.wikipedia.org/wiki/Type-I_superconductor
16.) https://en.wikipedia.org/wiki/Type-II_superconductor
17.)https://www.reddit.com/r/Physics/comments/bsxmoc/iisc_team_confirms_breakthrough_in/
18.) https://arxiv.org/ftp/arxiv/papers/1807/1807.08572.pdf